PROCESS CONTROL
By Ren Margolis-Dubow, Industrial Maintenance Lead, Rader Farms
Control, Monitoring, and Validation of Fruit Chemical Washing and Sanitizing Processes
Since different foods require significantly different wash methods, system design and sanitation process guidelines must be carefully considered
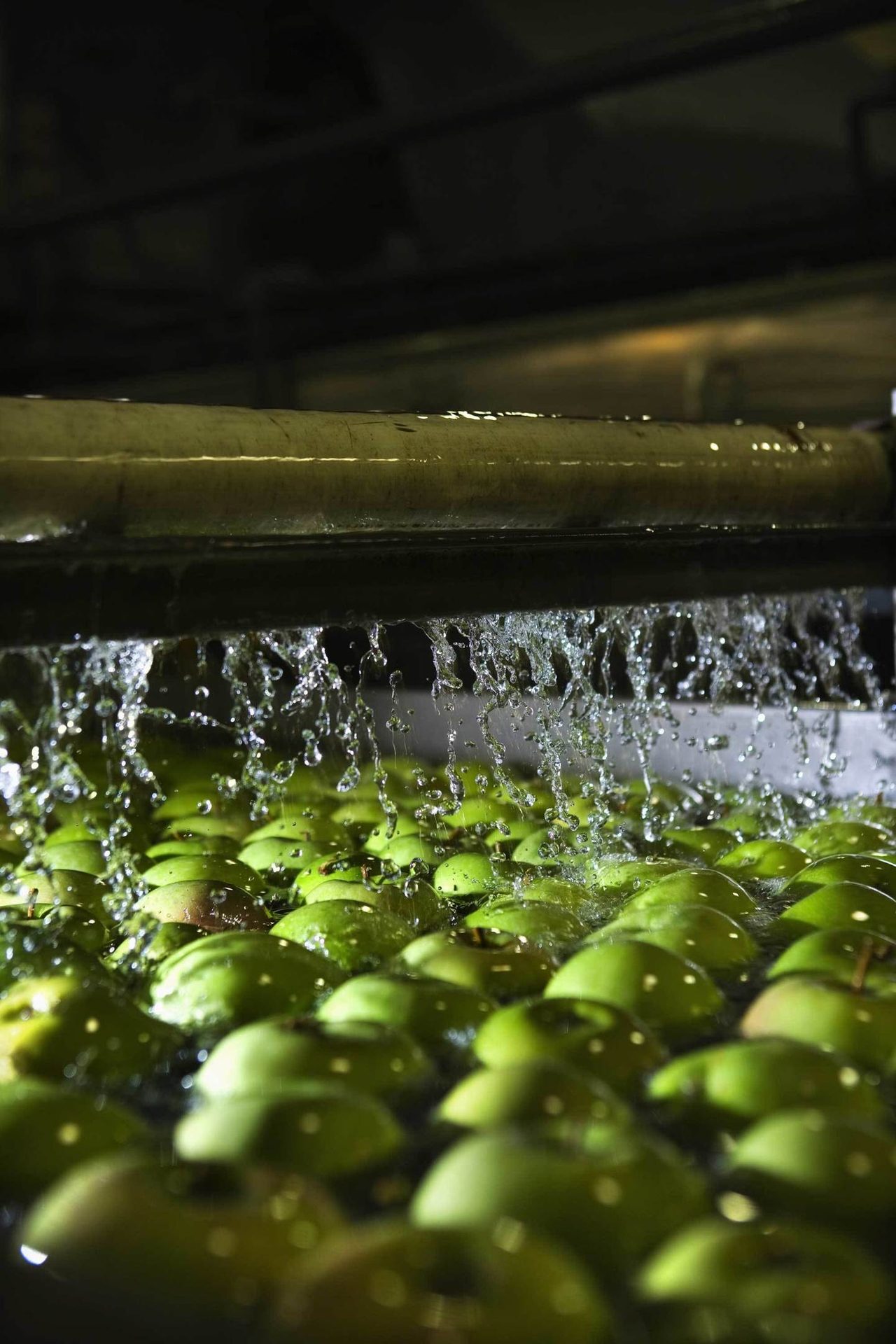
Image credit: Jupiterimages/Stockbyte via Getty Images
SCROLL DOWN
Critical food safety decisions are made every day by food processing employees from production workers to management. The ultimate goal is to provide safe and reliable products that meet consumer demand. In an increasingly automated world, empowering employees to make decisions based on real-time data is one of the most important factors in ensuring security and productivity. Continual improvement and learning should be baked into process development.
Whether establishing new processes or improving existing ones, clear and readily available frameworks help employees understand the dynamic criticality of process control points. There is limited information on establishing these systems, with much of the knowledge base existing in trade secrets. If the ultimate goal is total food safety, then it is paramount to establish industry standards that are clearly articulated and widely accessible.
Let us take the example of water use for produce cleansing. Significant evidence exists to support the washing and sanitizing of fruits, from apples to grapes to raspberries. At the same time, water is extremely effective at transmitting contaminants to fruit and vegetables, and has been linked to a number of pathogen outbreaks. Thus, wash water itself can pose a significant hazard. Since different foods require significantly different wash methods, system design and sanitation process guidelines must be carefully considered. In an effort to move toward clear, shared industry standards, this article details methodologies for wash water and sanitizer application in food processing facilities.
Establishing Control Points
Before jumping into design and application, it is good to start by looking at the relevant standards and regulations. In the realm of agricultural and processing water use, both Environmental Protection Agency (EPA) and Food and Drug Administration (FDA) guidelines come into play. Under the Food Safety Modernization Act (FSMA), FDA has issued regulations around produce safety. Excerpts from the two relevant sections appear below.
Referred to as "The Produce Rule," the first section is Standards for the Growing, Harvesting, Packing, and Holding of Produce for Human Consumption.1 This applies to raw agricultural commodities (RACs) that are intended for direct consumption.
Referred to as the "Preventive Controls Rule," the second section is Current Good Manufacturing Practice, Hazard Analysis, and Risk-Based Preventive Controls for Human Food.2 This section describes guidelines for otherwise processed produce. The preventive controls rule applies to facilities but not farms. Preventive controls must be based on scientific and technical information and systematically validated for efficacy.
To adhere to the Preventive Controls Rule, it is important to establish effective and validated systems. We have done this in my organization, and I share it here in the spirit of starting a public conversation to improve systems in food production facilities. The general steps are as follows:
- First, do initial testing and documentation before planning and constructing a system.
- Second, take samples of the product and analyze for contaminants, including biological, chemical, physical, and allergenic. Each type of contaminant requires an individual control methodology.
- Next, document this methodology thoroughly for later comparison testing.
- Finally, track results to be able to communicate the validity and effectiveness of such systems.
Many regulations address the types and concentrations of sanitizers. Different regulating agencies govern the use of sanitizers based on the process stage and which sanitizers are used. Figure 1 illustrates which agencies are responsible for regulating sanitation at different stages. It is easier and simpler, from a regulation standpoint, to wash and apply while the product is still a RAC. As long as sanitizer concentrations are kept within a certain range, sanitizers can be assumed to not count as an ingredient when used before the product is fully processed.
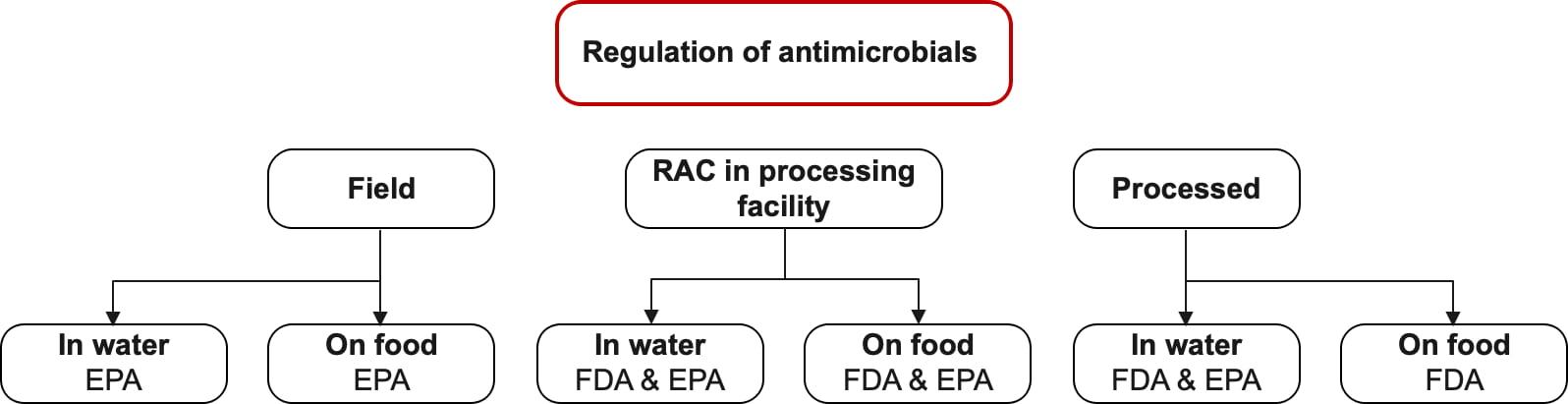
FIGURE 1. Agency Responsibility for Regulating Sanitation at Different Stages
Produce Sanitizing Methodology
The primary purpose for sanitizers in wash water is preventing cross-contamination. Water is extremely effective at spreading contamination between products. Fresh produce is typically the largest source of foodborne illness outbreaks, often through pathogens such as Escherichia coli, Listeria monocytogenes, and Salmonella. However, there is a growing trend of outbreaks being traced to processed fruits. Farm- and transport-level pathogenic prevention only goes so far due to the open nature of farming. Removal of hazards at the food processing site is important to consider when developing a multi-system plan of contaminant control. Although it may be counter-intuitive, washing should be viewed as a high-risk point of potential contamination. Numerous new options are available that allow sanitizing without washing; however, since many facilities still wash produce, this article will focus on the methods to best control pathogens within a wash water environment.
A limited number of scientific studies specifically address wash water sanitizing. Available related evidence is compiled here from multiple sub-sources to create general guidelines. Four chemical types are the focus of most research: chlorine in the form of calcium or sodium hypochlorite (Cl), peracetic acid (PAA), chlorine dioxide (ClO2), and ozone (O3).
Chlorine is commonly used as a wash water sanitizer in the processing industry due to its low cost, wide availability, and proven effectiveness. It works by causing oxidative damage to cell walls and disrupting key enzymes needed for pathogen growth, and has no residual sterilizing effect after application. However, appropriate concerns have been raised about the harmful disinfection byproducts (DBPs) produced in sub-reactions when chlorine interacts with organic matter. These sub-reactions result in significantly lowered concentrations after a relatively short exposure time. In a high-flow system with high organic matter load, a large amount of chlorine would need to be dosed to maintain sanitizing potential and will produce a significant amount of DBPs. There is carcinogenic potential in these byproducts, which led EPA to establish limit guidelines for several DBPs, including trihalomethanes (THM) and haloacetic acids (HAA). Given the potential hazards of chlorine with organic matter, other options should also be examined.
There is growing support for peracetic acid (PAA) for multiple reasons. First and foremost, it produces significantly fewer harmful byproducts than chlorine and breaks down more slowly when exposed to organic matter. The mechanism of action for PAA is oxidation, and the sanitizing effectiveness of peracetic acid is largely pH independent. Additionally, PAA continues to sterilize for hours after application, even when frozen. These are distinct advantages in efficiency and healthfulness, compared to chlorination. Other, newer technologies have emerged that should also be considered.
Non-chemical cold plasma is a new and highly effective technology that uses energetic, reactive plasma gas at a relatively low temperature to deactivate a wide range of pathogens. Currently, however, the process is complex and expensive, and it does not remove other types of contaminants. Also, the impact on food taste and nutrients has not been studied as thoroughly to date as other methods.
Other notable approaches for disinfection include ultraviolet (UV) sterilization and ozone. The contact time between produce and sanitizer must also be considered. For chemical-based wash water, a minimum of around 20 seconds of exposure time is recommended for most applications. Multiple methods can be integrated for more effective, broad-spectrum sterilization (Table 1).
TABLE 1. Sanitizer Methods and their Sensitivity to Organic Matter
Validation and Documentation
If the washing system is being considered as a control point with a need for high levels of measurable efficacy, then quantitative metrics must be established. Guidelines3 were published by Gombas et al. in 2017 in the Journal of Food Protection to validate control of cross-contamination during washing of fresh-cut leafy vegetables. Recognizing that fruits are their own category with separate issues that are characterized by a lack of available research, fresh fruit washing makes a good starting point to explore.
Gombas et al.3 outline three options for validation for fresh vegetables:
- Use a test surrogate for the microbial hazard and establish clear proof that the hazard is prevented or reduced by the sanitizing wash
- Use sensors to ensure that critical levels of sanitizer are maintained, and focus on worst-case scenarios
- Validate the placement of sensors and ensure that a critical level of sanitizer is maintained, regardless of operating condition.
All of these are good ways of validating efficacy. In an ideal scenario, all three methods of validation would be used. Here, we will focus on methods two and three. For validation of sanitizer concentration, several sub-categories of testing methods can be employed:
- Manual chemical testing by test strips or wet kit and manual dosing. The advantage here is low cost and instantaneous results. Samples should be taken at a minimum hourly interval.
- Partially automated testing with sensors and manual or flowrate-controlled dosing. Advantages include ease of setup and ability to store continuous monitoring data. However, any deviation in sanitizer levels will need to be manually corrected.
- Fully automatic testing with sensors and remote-control dosing. Here, pay attention to end-to-end automation of the process to reduce the possibility of human error and ensure high levels of consistency. Deviation alarms, auto purging, and line stop can also be incorporated.
Regardless of the sanitizer testing and application method used, hazard testing should be conducted on a regular basis, such as at the beginning of every shift. The most thorough method is to test on the production line before washing, in the wash water, and post washing. This can and should be done in the initial study to determine the sanitizer levels and contact time needed with the fruit. Typically, post-wash testing on a regular basis would be sufficient to prove that microbial levels are being controlled, as long as the dosing and level control systems are functioning properly and are consistent. However, any deviation in the wash system must be thoroughly documented and retested to ensure that levels return to normal before production continues. If this happens, a failure analysis should be conducted, documented, and incorporated into the maintenance program to prevent further issues.
The distinction between validation and verification is also important. Validation focuses on determining if implemented control measures are effective. Verification is the process of ensuring that validation is carried out in accordance with the hazard control plan and is providing usable results.
While it is easy to implement, using a simple stop-or-go response method for elevated contamination levels is overly simplistic and does not aid in creating a culture of prevention. Validation should focus on driving process and control improvement, rather than just generating raw data.
Which testing methods are selected and implemented should also be given careful consideration. The testing methods are the only conduit to proper validation. Without proper validation, there is little to suggest that a given plan is effective in reducing and controlling hazards. Testing guidelines should be not only clear, but also meaningful in their approach to providing data that is actionable and easily understood. Training of quality assurance staff is key in ensuring that hazards are recognized and understood. Common problem areas can be identified quicker and more accurately if staff know what to look for and why. With this heightened observation and knowledge, staff can communicate more concretely with supervisors, who can then develop more effective procedures for controlling identified hazards. It can also benefit processors to think outside of the scope of a HACCP program to include whole-system analysis. There is often insufficient data to truly determine the likelihood and direct danger of a hazard. More often, there is a vague concept of "bad" or "good" results. Continuous analysis of consistent systems is the single most effective way of ensuring that the wash water itself does not become a hazard. For example, we know that maintaining the temperature of food can prevent pathogen growth, and we do not need to test every single product.
Swanson and Anderson4 offer helpful insight into the importance of in-process testing. In their 2000 article in the Journal of Food Protection, they explained that in-process testing "provides more information than finished product testing," but note that "attribute-based acceptance testing is fraught with sampling issues."4 They go on to describe some of the high stakes related to sampling:
Increasing the number of samples can reduce the probability of accepting a defective lot, but it cannot eliminate it entirely. For example, if 10 percent of the sample units in a lot contain the hazard, the lot will be accepted six of ten times when five samples are tested for the hazard. If 30 samples are tested, it will be accepted about five in 100 times and less than one in 100 times if 60 samples are tested. Having Salmonella in 1 percent of a ready-to-eat product is obviously unacceptable.4
Since we know water is excellent at transmitting contamination, we now see it is critical to identify and eliminate the specific hazards in wash systems upfront (Figure 2).
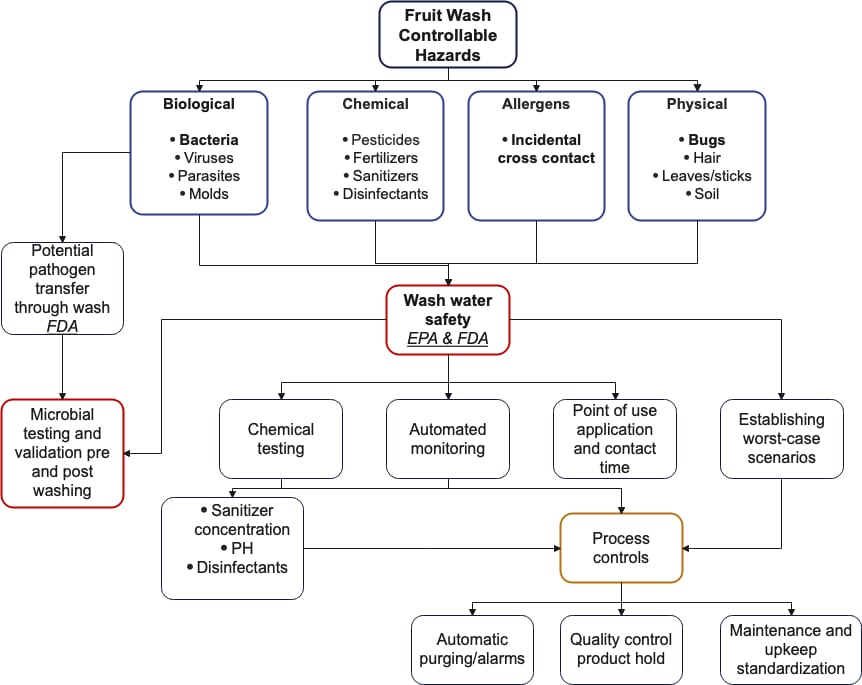
FIGURE 2. Controllable Hazards in Fruit Water Wash Operations
System Design
Given all the potential hazards and the difficulties inherent in testing, as many parts of the system must be secured as possible. Sanitizer concentration and exposure time are critical components that can be controlled once they are identified. Dosing and maintaining acceptable sanitizer concentration and exposure time may also be the most difficult parts to get right in washing systematization. With dynamically changing organic loads and high throughput, careful chemical monitoring is needed. One historical go-to has been to nuke the water with high doses and do a post-rinse; this is an antiquated method with less consistency and higher potential for harmful byproducts than measured dosing and controlling application time.
Many commercially available sensors for monitoring sanitizer concentration exist, and they are fairly affordable. A number of methods for actual washing are also available, from flume systems to float tanks to simple spray bars. The single most effective application method is full immersion of product with water agitation. With this method, high overall exposure to sanitizing agents and long contact times improves chances of successful sanitization. This method also provides the greatest chance for cross-contamination if sanitizer concentrations have not been met, since the water can carry contamination between products.
In this article, we will focus on dosing systems and maintaining sanitizer concentration since they are critical for preventing hazards. There are three common dosing system layouts (Figure 3):
- Inline dosing: The most straightforward system, this setup features a water source, inline chemical doser, and monitoring sensor that goes directly to the point of use. This is the easiest to setup and has the fastest response time to inputs; however, if concentrations are out of specification, this will show up very quickly. Integrating some sort of automated line stop or alarm is recommended.
- Recirculated system: Borrowed somewhat from swimming pool chlorine dosing designs, the recirculated system can be effective if very high volumes or dynamically changing organic loads are present. A high-flow recirculated line feeds the point of use and circles back into a holding tank. An optional low-flow recirculated line can increase reliability due to the high throughput, allowing for more active monitoring. When chemical is dosed in a high-flow recirculated line, it can potentially waste a significant amount of water. This can be remedied, however, if the point of use is tied back into the low-flow recirculated line.
- Reuse system: Reuse systems are common on less critical applications, such as tray, lug, and pallet washing. It typically involves multiple holding tanks that circulate the water through the point of use and back into one tank at a time while the other tank is filling. A secondary doser can be fitted on the tanks to maintain level, but if the tanks are switched often enough (such as hourly), it is typically more feasible to maintain proper sanitizer concentrations.
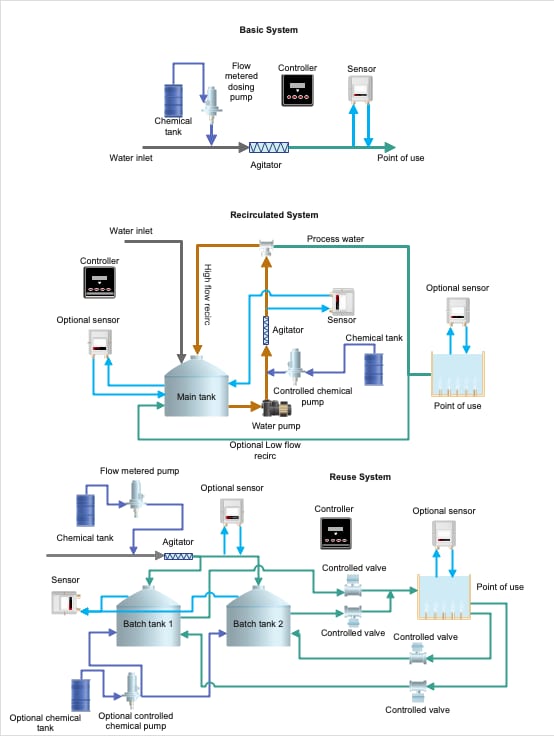
FIGURE 3. Common Dosing System Layouts
Takeaway
Given all the potential hazards in fruit washing and the intricacies of the different approaches to mediating or mitigating these hazards, it becomes clear that establishing a hazard-controlled, safe wash water system can be time-consuming and require investment on both the operations and quality side of processing. To establish such a hazard-controlled wash-water system, it is critical to know the scope of the targeted hazards. The process for doing so can be summed up in four steps:
- Hazard analysis: Identify presented and predicted hazards, and test for pathogenic contamination.
- Development of controls: Is washing required? If it is, how can contamination be prevented from entering the wash water itself?
- System implementation: Consult all involved parties on what kind of system will work best for the given application. Not only supervisors, but also line workers, will have important information for such a discussion.
- Post-install validation: Test for system efficacy. Is the sanitizing process working effectively? If not, what can be changed?
A system is only as strong as its weakest link. After the entire system is designed and ready to be implemented, create a training program to educate employees on the identification and communication of hazards. Everyone who works in a processing facility should be aware of potential hazards and how to prevent them. With a whole-team, whole-process approach, we can meet regulations, minimize food contamination, and maintain business profitability.
Resources for More Information
One potential resource for designing high-flow chemical dosing systems is the American Water Works Association (AWWA). AWWA offers a manual providing detailed information on the design and operation of chemical feed systems for water and wastewater treatment, including guidance on the selection and sizing of equipment, the calculation of feed rates, and the control of chemical dosing.
Additionally, industry associations and trade organizations can be a useful source of information on the design of high-flow chemical dosing systems. For example, the National Association of Chemical Distributors (NACD) provides a range of resources and guidance on the safe handling and use of chemicals in industrial applications, including information on chemical dosing and handling.
References
- U.S. Food and Drug Administration (FDA). Standards for the Growing, Harvesting, Packing, and Holding of Produce for Human Consumption. November 27, 2015. https://www.federalregister.gov/documents/2015/11/27/2015-28159/standards-for-the-growing-harvesting-packing-and-holding-of-produce-for-human-consumption
- FDA. FSMA Final Rule for Preventive Controls for Human Food: Current Good Manufacturing Practice, Hazard Analysis, and Risk-Based Preventive Controls for Human Food. July 10, 2020. https://www.fda.gov/food/food-safety-modernization-act-fsma/fsma-final-rule-preventive-controls-human-food
- Gombas, D., Y. Luo, J. Brennan, et al. "Guidelines to Validate Control of Cross-Contamination during Washing of Fresh-Cut Leafy Vegetables." Journal of Food Protection 80, no. 2 (February 2017): 312–330. https://pubmed.ncbi.nlm.nih.gov/28221982/.
- Swanson, Katherine M. J. and J. E. Anderson. "Industry Perspectives on the Use of Microbial Data for Hazard Analysis and Critical Control Point Validation and Verification." Journal of Food Protection 63, no. 6 (2000): 815–818.
Ren Margolis-DuBow is an industrial maintenance professional with a background in food production and a deep interest in systematization, standardization, and automation.